Medial Radiation Physics
Medical Radiation Physics

Radiotherapy is a treatment modality where ionizing radiation is used to kill cancer cells. Radiotherapy most often uses x-rays (photons), but proton therapy is a novel and technologically advanced radiotherapy used for some patients. Optimal radiotherapy requires integration of state-of-the art medical imaging technologies.
Our research is based on developments within the field of advanced imaging technology (functional MRI and PET). We focus particularly on how we can integrate quantitative measures from these technologies in further optimization of radiotherapy of cancer. By imaging and understanding the cancer biology and individual radiation sensitivity, we aim to develop new concepts within image-based biologically adaptive radiotherapy. Our research is performed in close collaboration with St. Olavs hospital, but also together with several of the other national university hospitals as well as international partners.

Research topics
Quantitative MRI
Radiotherapy is important in multimodal treatment of solid cancer, often in combination with other treatments such as chemotherapy, immunotherapy and surgery. However, to deliver precision radiotherapy optimised for each patient, the initial diagnostics is essential. Through the last decade, the use of medical imaging, and especially MRI, which provides images with excellent soft tissue contrast, has become a central part in diagnostics. Compared to tissue biopsies, often giving a snap-shot of a heterogeneous tumour, MRI provides whole-tumour assessment as well as possibility for repeated evaluations. However, today, the full potential of MRI is not exploited. Whereas MRI is known to visualize the anatomy and evaluate local disease extent, technological advances have given many new opportunities, including the possibility to also quantitatively measure tumour biological features which may be directly used to optimise treatment.
We work on developing new therapeutic frameworks for non-invasive personalised medicine by utilising 4D and 5D functional MRI techniques to map the vascularity, oxygenation and radiation sensitivity in malignant tumours. Our aim is to integrate quantitative MRI in improving stratification of patients to the optimal, personalised treatment resulting in improved tumour response and prolonged survival, reduced side-effects and less personal and societal burden.
- Comparison of Intravoxel incoherent motion imaging and multiecho dynamic contrast-based MRI in rectal cancer.
- Dynamic multi-echo DCE- and DSC-MRI in rectal cancer.
- Personalized radiotherapy: concepts, biomarkers and trial design.
Our research has recently resulted in development of a new multi-channel MRI software where multi-echo 4D and 5D data sets can be processed.
Functional 5D MRI of rectal cancer vascularity. Top right: Kinetic curves reflecting two different dynamic responses acquired with the multi-echo split-dynamic MRI method following injection of contrast agent (1) the change in longitudinal relaxation rate (R1) and (2) the change in transversal relaxation rate (R2*). Utilising these kinetic curves in a voxel-wise manner allows estimation of tumour maps of oxygen- and vascularity measures. Top left: Anatomic image of rectal cancer. Lower row: tumour maps of perfusion (Ktrans parameter), blood volume and R2* peak (max amplitude), an oxygenation-related parameter.
MR-only radiotherapy
During the last years, the use of MR imaging in radiotherapy planning has increased rapidly due to its superior soft tissue contrast. MR images allow a more accurate delineation of the tumour and organs at risk, compared to CT which traditionally has been considered as the primary modality in radiotherapy. As the linear accelerator has a flat table, MR scanners used in radiotherapy also needs to have flat table overlay to be able to image the patient in treatment position. The scanner should also have a bore large enough to make room for the radiotherapy specific equipment, such as immobilization devices and coil bridges.
The coil bridges are used to create a gap between the patient and the receiver coil to avoid deformation of the patient. But still the gap should be minimal since the signal decreases with increasing distance. The addition of an external laser bridge is needed to position the patient as precisely as possible. The voxel intensity of CT images provides direct information about the electron density of the tissue, providing information about how photons will interact with the tissue. This data is needed to accurately calculate the resulting radiation dose.
One challenge is that electron density is not retrieved in MR, where the image intensities correspond to proton density and relaxation. It is, however, possible to generate synthetic CT images based on the MR images. We are working on developing a workflow for the use of MR-only in radiotherapy. Within this we also collect quantitative MR data and match this with the delivered radiation dose in 3D volumes. This enables us to model how quantitative MR measures reflecting cancer biology and radiation sensitivity are connected to the patients’ normal tissue toxicity and tumour responses.
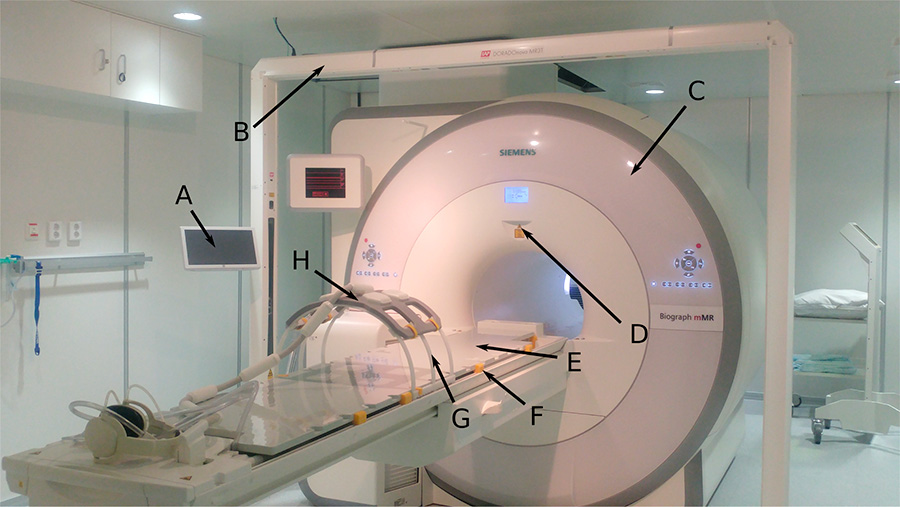
The PET/MR scanner at St. Olavs hospital (Siemens Biograph mMR) with equipment needed for MR-based radiotherapy. A) Tablet to control the laser bridge, B) laser bridge, C) the magnet, D) scanner isocenter-location laser, E) Mediboard flat table overlay, F) blocks used to secure the flat overlay on top of the standard curved patient table, G) torso coil holder, H) MR receiver coil placed on the coil holder.
Artificial intelligence in radiotherapy
Artificial intelligence is rapidly integrated into several steps of the radiotherapy workflow, such as target and tissue segmentation, treatment planning, radiotherapy delivery and treatment response assessment. We currently have ongoing activities in developing tools for automatic tumor segmentation in CT, MR and/or PET images by using machine learning and deep learning. In particular we investigate how functional MRI and PET, reflecting cancer biology, vascularity and other characteristics of the tumour microenvironment, can help to improve the segmentations compared to using only the anatomic imaging. Such tools are necessary both for the radiotherapy planning process and for retrieval of quantitative tumour measures for prognostic and predictive purposes.
Together with St. Olavs hospital we investigate novel machine learning methodology for automatic treatment plan generations and deep-learning organ segmentation using the treatment planning system RayStation.


Proton therapy
Proton therapy is a technologically advanced treatment modality that uses a beam of high-energy protons to irradiate the tissue. Compared to the conventional photon-based radiotherapy the proton therapy benefits from delivering a more precise radiation dose with lower dose to surrounding critical organs (see figure). In Norway we will soon get two proton therapy centers both for treatment of patients and for research.
Tissues respond differently to photon-based radiotherapy and proton therapy. We investigate how we can use functional imaging (MR and PET) to characterize the malignant tumour and organs at risk and use the information to optimize the delivery of proton therapy. For instance, how to adapt proton therapy according to tumour hypoxia, which increases radiation resistance and requires increased radiation doses compared to well oxygenated tumours, is still unclear. Furthermore, more studies are required to identify how we best select patients benefiting from proton therapy.
Treatment with photons
Treatment plan with protons
Figure: Example on difference in radiation dose distribution in photon-based radiotherapy and proton therapy for patient with lung cancer.
Hybrid PET/MR
The molecular and physiological data obtained with the unique combination of simultaneous PET and MRI acquired in a hybrid PET/MR scanner creates a basis for new and improved understanding of cancer development and therapeutic responses. We participate in the Norwegian Nuclear Medicine Consortium project 180N and are responsible for a work package on the use of PET/MRI for radiotherapy purposes. This is a multicenter project with clinical studies in head and neck cancer and in prostate cancer performed together with colleagues in Tromsø and Bergen. We aim to use hybrid PET/MR to establish clinical workflow for PET- and MRI-based radiotherapy. By acquiring PET and MR images prior to and during radiotherapy we work on developing new concepts for image-based biologically adaptive radiotherapy, both based on state-of-the-art photon-based radiotherapy and also proton therapy, which soon will be available for cancer patients in Norway. Our aim in this strand of our research is to contribute towards further developments of personalised high-precision radiotherapy resulting in improved outcome, reduced side-effects and better quality of life for cancer patients.
New PET tracers
Tumour hypoxia (low tissue oxygen levels) is an important adverse feature of the tumour microenvironment that leads to more aggressive disease and overall significantly worsen cancer prognosis. In addition, tumour hypoxia is also the major factor that causes external radiotherapy resistance. To date, no effective method has been fully established to precisely detect tumour hypoxia. Studies using 64Cu-based tracers in PET imaging have demonstrated promising results to detect tumour hypoxia with selective retention of 64Cu in hypoxic regions of the tumours. In addition, it has been demonstrated that high dose of 64Cu nuclear medicines may be used for internal radiotherapy through what is called Auger electrons. However, to establish a consistent imaging and treatment systems, it is necessary to identify the best suited tracers. Recent studies have also raised questions on the validity of the previously proposed copper uptake mechanism in the tumour, which appears to be more copper-related instead of ligand-related, thus requiring more in-depth investigations.
We are currently performing a project together with the Department of Nuclear Medicine at St. Olavs hospital where we aim to develop 64Cu-based radiopharmaceuticals for the imaging of hypoxic tumours using PET. A secondary aim is to investigate the possibility of using such tracers at high dose for internal radiotherapy. Thirdly, we also want to improve our understanding of the copper uptake mechanism in hypoxic tumours, addressing the discrepancy found in recent evidence compared to the previously proposed mechanism. The result of this project is clinically relevant and aimed to improve the treatment outcome of a large group of patients using advanced imaging technology and may help to develop individualized high-precision cancer treatment.

Group members
-
Signe Danielsen Adjunct Associate Professor
+47-73593392 +4793626293 signe.danielsen@ntnu.no Department of Clinical and Molecular Medicine -
Kajsa Maria Linnea Fridström
kajsa.m.l.fridstrom@ntnu.no Department of Physics -
Guillermo Garrido Hernandez
guillermo.g.hernandez@ntnu.no Department of Physics -
Endre Grøvik Researcher
endre.grovik@mn.uio.no Department of Physics -
Marte Kåstad Høiskar PhD Candidate
marte.k.hoiskar@ntnu.no Department of Physics -
Franziska Knuth PhD Candidate
franziska.h.knuth@ntnu.no -
Anne Beate Langeland Marthinsen Associate Professor
+47-73413094 anne.b.marthinsen@ntnu.no Department of Physics -
Rene Mario Winter Asst. Professor / Researcher
+47-73592045 rene.winter@ntnu.no Department of Physics