Multi-phase and free-surface flows - Thermo fluids - Department of Energy and Process Engineering (EPT)
Multi-phase and free-surface flows

Individual research areas
Non-contact manipulation of micro- and nano-size particles plays a leading role in modern microsystem technology due to its relevance in diverse engineering and scientific areas such as material science, biomedical and clinical diagnostics. Acoustic fields can induce forces by scattering of the acoustic waves on individual particles or by the attenuation of the acoustic field in the fluid leading to flow streaming, opening to potential applications of indirect manipulation of particles, mixing, lysis of cells, etc. However, the control of the position, orientation and scale of the acoustic forces inside the bulk liquid, in addition to thermal management and scaling of the actuation of the acoustic forces to large volumes in the millilitre range remain an unmet challenge.
Focus of our work
Our research focuses on the design and fabrication of acoustic-based devices, and the study of acoustic induced flow streaming and acoustic radiation forces. We aim to design acoustic based operators for flow and particle actuation, including both synthetic particles and cells.

Methods
We fabricate the SAW devices at the NTNU NanoLab cleanroom using Laser and Electron Beam Lithography combined with metal deposition by E-beam for the fabrication of the interdigital electrodes. The devices are characterized using Scanning Electron Microscopy and optical microscopy, electrically by a network analyser, and thermally with an infrared camera.
Flow visualization experiments are conducted using a fluorescence inverted microscope, a digital holographic microscope, and a micro-PIV-LIF system. We use functionalized synthetic particles and human-cells. The facilities include a biosafety level 2 cabinet, cell incubator, cell counting, Qubit fluorometer for DNA and protein quantification, E-Gel Power Snap electrophoresis, 2100 Bioanalyzer and Multiskan™ FC Microplate Photometer. The studies are complemented with analytical and numerical studies.
For more information, please contact Maria Fernandino or Carlos Dorao.
More than 50% of anthropogenic CO2 is stored in the oceans, but we do not fully understand the processes by which this transport happens. The problem is typically framed as a chemistry problem relating to salinity and partial pressures, but at NTNU we have demonstrated that the rate of gas transfer from air to water can change by more than 30% by only changing the turbulence characteristics under the surface.
Given that the transfer rate of gases in climate models are typically set as a constant or a very simple parameterisation, understanding these processes more fully can have significant ramifications for our understanding and modelling of the climate system, but also for industrial and ambient applications of carbon capture and sequestration.
Focus of our work
We focus on removing the chemistry elements from the gas transfer problem so that we can understand how the fluid mechanics influences the processes. We aren’t saying chemistry isn’t important; we are just trying to isolate the fluids processes.
By keeping the chemistry the same, we can then adjust the bulk flow and turbulence in either the air, the water, or both, so that we can see how the gas transfer rate is influenced. Such a set-up also allows us to look at other physical processes in the systems such as momentum transport.
Methods
Through the ERC Starting Grant GLITR (PI: Jason Hearst) we investigate these problems experimentally in the lab. We have two key facilities in the Fluid Mechanics Laboratory at NTNU that allow us to manipulate the turbulence intensity, length scales, and flow profiles in the air and water separately. These are called active grids. We then use high-fidelity laser-diagnostics to measuring the velocity fields in both phases (air and water) and we can simultaneously measure the dissolved gas concentration in the water using a laser-induced fluorescence technique.
By doing these experiments, we are able to both measure how turbulence changes the process of gas transfer at an air-water interface on average, but also observe the active mechanisms that are responsible for these mean changes.
For more information, please contact Jason Hearst.
Numerical simulation of two-phase flows presents challenges such as representation of moving interfaces, fluid properties changing abruptly in a short length scale, surface tension effects difficult to capture numerically and the multiscale problem related to fluid-solid interaction near contact lines. Phase change phenomena such as evaporation and condensation add complexities to the problem.
Focus of our work
Our research focuses on diffuse interface methods for studying interfacial flows with phase change. In particular, we work with the Navier-Stokes-Korteweg model, which considers the fluid density as the phase-field parameter for identifying the liquid and vapor phases.
The model handles compressible two-phase flow dynamics through van der Waals’ gradient theory along with a non-convex equation of state, which endows capillarity effects and phase transformation without ad-hoc assumptions on the gas-liquid interface. With this model we investigate heat and mass transfer processes during evaporation and condensation of droplets, trying to understand how local changes of the interface curvature and local thermodynamic conditions affect the phase change phenomena.

Methods
The Navier-Stokes-Korteweg model is solved numerically by our in-house Least Squares Spectral Element Method simulation code. The code is a multi-purpose simulation platform including high order C0 Lagrangian interpolant polynomials, and C1 third order Hermite functions extended with bubbles functions. A non-conforming adaptive mesh refinement strategy using mortar elements is used to achieve the necessary resolution at fluid interfaces. The final system is solved by a parallel element-by-element CG or alternative direct solvers.
For more information, please contact Maria Fernandino or Carlos Dorao.
Efficient heat dissipation in high heat flux systems such as microelectronics, defence systems, and nuclear reactors is essential in order to prevent performance degradation and avoid possible system failure. For instance, it is important to prevent the breakdown in heat transfer performance, termed a "boiling crisis", that can occur when a solid surface becomes blanketed with vapour, or the "Departure from Nucleate Boiling" (DNB) phenomena cause by merging of bubbles generated at a solid surface during boiling.
The breakdown in heat transfer performance can also be caused by the occurrence of two-phase flow instabilities the density wave oscillations (DWO), pressure drop oscillations (PDO), and thermal oscillations (THO). These phenomena are of interest for the design, operation and safety of boiling nuclear reactors and refrigeration plants. Unfortunately, understanding of these phenomena is still lacking. This lack of understanding constitutes many times a bottleneck for the optimization of these systems and for the development of novel technologies.

Focus of our work
Our research focuses on studying the heat transfer mechanisms during flow boiling inside a heated pipe during convective and nucleate flow boiling. We aim to identify the heat transfer mechanisms governing these two cases and the transition between them. In addition, we study the mechanisms leading to the occurrence of different types of two-phase flow instabilities, the coexistence of different instabilities, the characteristics of the instabilities in terms of amplitude and period, and whether they can lead to heat transfer deterioration mechanisms.

Methods
We use our Two-Phase Flow Instability facility specially designed for studies of flow boiling inside a heated pipe under transient operations. The facility includes a horizontal and a vertical test section, and a 5-parallel tubes horizontal test section for studies of flow maldistribution effects. High-speed laser and camera systems are used for studies of the flow structure.
For more information, please contact Maria Fernandino or Carlos Dorao.
For more information, please contact Simen Ellingsen.
Wetting is a common phenomenon in a variety of practical domains including self-cleaning and anti-icing surfaces, heat management in high heat fluxes systems, painting and coating, corrosion of turbine blades and aircrafts, separation and oil repellency, among others. Understanding the mechanisms controlling wetting and droplet dynamics is essential for the design of tailored, durable and optimal surfaces for these applications. However, wetting dynamics is controlled by complex solid-liquid interfacial interactions occurring in scales raging from molecular to millimetric sizes. This multiphysics and multiscale behaviour, in addition to the short timescale involved, constitute a challenge for the investigation and understanding of the phenomena involved.
Focus of our work
Our research focuses on tunning the solid-liquid interfacial interactions by designing and fabricating micro- and nano-structured surfaces. These surfaces provide us with a tool for studying the mechanisms controlling wetting and droplet dynamics. We study the forces, velocity, and the singular flow near the vicinity of contact lines with the aim of identifying the mechanisms governing the dynamic wetting process.

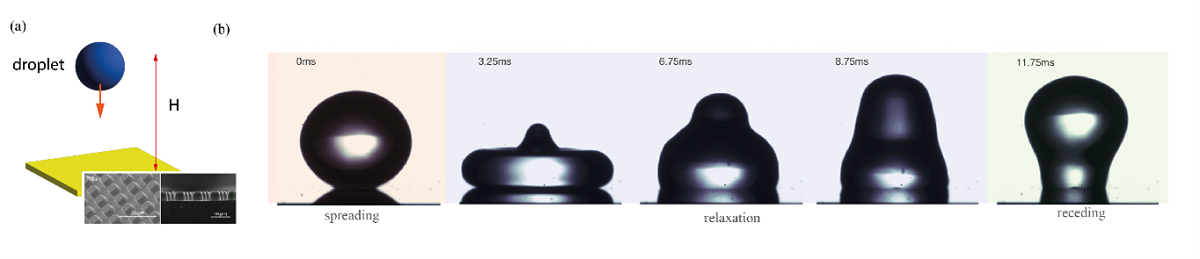
Methods
We fabricate the surfaces in at NTNU NanoLab cleanroom, using micro-/nano-fabrication techniques, such as Laser and Electron Beam Lithography combined with dry and wet etching. These surfaces and their wetting properties are characterized by Scanning Electron Microscopy and optical tensiometer. Our experimental methods for studying wetting dynamics include high-speed cameras and laser systems for studying droplet impact dynamics, a micro-PIV-LIF system used for studying the flow and temperature field inside sessile droplets, high speed infrared thermography for observing the temperature field, digital holographic microscopy for studying the dynamics of contact lines, and long working distance microscopy for studies related to evaporation and condensation. The experimental studies are combined with analytical and numerical methods for helping in the interpretation of the phenomena.
For more information, please contact Maria Fernandino or Carlos Dorao.
People at the research area
-
Carlos Alberto Dorao Professor
+47-73598462 carlos.dorao@ntnu.no Department of Energy and Process Engineering -
Simen Ådnøy Ellingsen Professor
simen.a.ellingsen@ntnu.no Department of Energy and Process Engineering -
Maria Fernandino Professor
+47-73595352 maria.fernandino@ntnu.no Department of Energy and Process Engineering -
R. Jason Hearst Professor
+4791359427 jason.hearst@ntnu.no Department of Energy and Process Engineering